This story is excerpted from THE WEIGHT OF NATURE: How a Changing Climate Changes Our Brains, available April 9, 2024, from Dutton, an imprint of Penguin Publishing Group.
Imagine you are a clown fish. A juvenile clown fish, specifically, in the year 2100. You live near a coral reef. You are orange and white, which doesn’t really matter. What matters is that you have these little ear stones called otoliths in your inner ear, and when sound waves pass through the water and then through your body, these otoliths move and displace tiny hair cells, which trigger electrochemical signals in your auditory nerve. Nemo, you are hearing.
But you are not hearing well. In this version of century’s end, humankind has managed to pump the climate brakes a smidge, but it has not reversed the trends that were apparent a hundred years earlier. In this 2100, atmospheric carbon dioxide levels have risen from 400 parts per million at the turn of the millennium to 600 parts per million — a middle‑of‑the-road forecast. For you and your otoliths, this increase in carbon dioxide is significant, because your ear stones are made of calcium carbonate, a carbon-based salt, and ocean acidification makes them grow larger. Your ear stones are big and clunky, and the clicks and chirps of resident crustaceans and all the larger reef fish have gone all screwy. Normally, you would avoid these noises, because they suggest predatory danger. Instead, you swim toward them, as a person wearing headphones might walk into an intersection, oblivious to the honking truck with the faulty brakes. Nobody will make a movie about your life, Nemo, because nobody will find you.
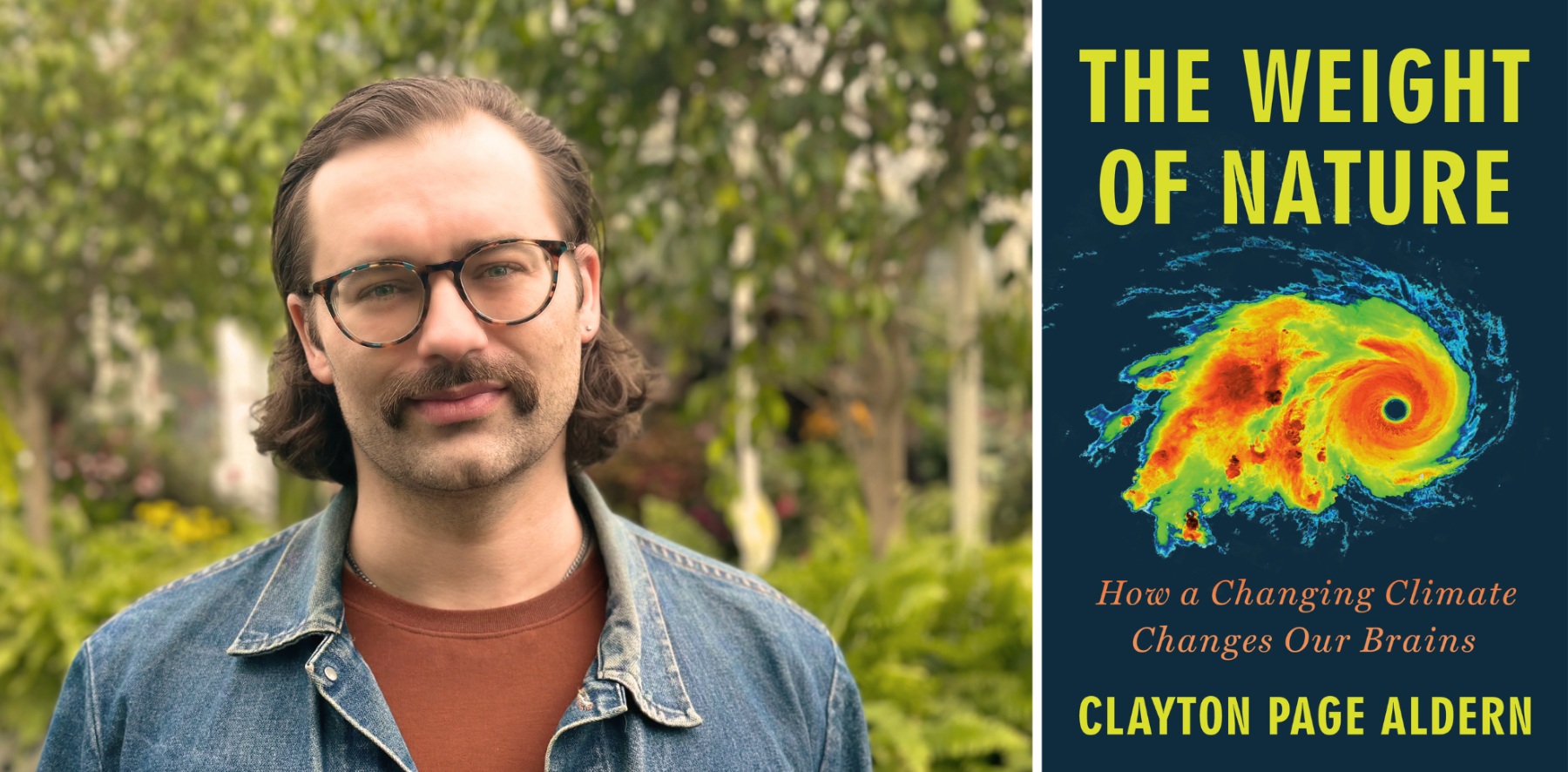
It’s not a toy example. In 2011, an international team of researchers led by Hong Young Yan at the Academia Sinica, in Taiwan, simulated these kinds of future acidic conditions in seawater tanks. A previous study had found that ocean acidification could compromise young fishes’ abilities to distinguish between odors of friends and foes, leaving them attracted to smells they’d usually avoid. At the highest levels of acidification, the fish failed to respond to olfactory signals at all. Hong and his colleagues suspected the same phenomenon might apply to fish ears. Rearing dozens of clown fish in tanks of varying carbon dioxide concentrations, the researchers tested their hypothesis by placing waterproof speakers in the water, playing recordings from predator-rich reefs, and assessing whether the fish avoided the source of the sounds. In all but the present-day control conditions, the fish failed to swim away. It was like they couldn’t hear the danger.
In Hong’s study, though, it’s not exactly clear if the whole story is a story of otolith inflation. Other experiments had indeed found that high ocean acidity could spur growth in fish ear stones, but Hong and his colleagues hadn’t actually noticed any in theirs. Besides, marine biologists who later mathematically modeled the effects of oversize otoliths concluded that bigger stones would likely increase the sensitivity of fish ears — which, who knows, “could prove to be beneficial or detrimental, depending on how a fish perceives this increased sensitivity.” The ability to attune to distant sounds could be useful for navigation. On the other hand, maybe ear stones would just pick up more background noise from the sea, and the din of this marine cocktail party would drown out useful vibrations. The researchers didn’t know.
The uncertainty with the otoliths led Hong and his colleagues to conclude that perhaps the carbon dioxide was doing something else — something more sinister in its subtlety. Perhaps, instead, the gas was directly interfering with the fishes’ nervous systems: Perhaps the trouble with their hearing wasn’t exclusively a problem of sensory organs, but rather a manifestation of something more fundamental. Perhaps the fish brains couldn’t process the auditory signals they were receiving from their inner ears.
The following year, a colleague of Hong’s, one Philip Munday at James Cook University in Queensland, Australia, appeared to confirm this suspicion. His theory had the look of a hijacking.
A neuron is like a house: insulated, occasionally permeable, maybe a little leaky. Just as one might open a window during a stuffy party to let in a bit of cool air, brain cells take advantage of physical differences across their walls in order to keep the neural conversation flowing. In the case of nervous systems, the differentials don’t come with respect to temperature, though; they’re electrical. Within living bodies float various ions — potassium, sodium, chloride, and the like — and because they’ve gained or lost an electron here or there, they’re all electrically charged. The relative balance of these atoms inside and outside a given neuron induces a voltage difference across the cell’s membrane: Compared to the outside, the inside of most neurons is more negatively charged. But a brain cell’s walls have windows too, and when you open them, ions can flow through, spurring electrical changes.
In practice, a neuron’s windows are proteins spanning their membranes. Like a house’s, they come in a cornucopia of shapes and sizes, and while you can’t fit a couch through a porthole, a window is still a window when it comes to those physical differentials. If it’s hot inside and cold outside, opening one will always cool you down.
Until it doesn’t.

Here is the clown fish neural hijacking proposed by Philip Munday. What he and his colleagues hypothesized was that excess carbon dioxide in seawater leads to an irregular accumulation of bicarbonate molecules inside fish neurons. The problem for neuronal signaling is that this bicarbonate also carries an electrical charge, and too much of it inside the cells ultimately causes a reversal of the normal electrical conditions. At the neural house party, now it’s colder inside than out. When you open the windows — the ion channels — atoms flow in the opposite direction.
Munday’s theory applied to a particular type of ion channel: one responsible for inhibiting neural activity. One of the things all nervous systems do is balance excitation and inhibition. Too much of the former and you get something like a seizure; too much of the latter and you get something like a coma — it’s in the balance we find the richness of experience. But with a reversal of electrical conditions, Munday’s inhibitory channels become excitatory. And then? All bets are off. For a brain, it would be like pressing a bunch of random buttons in a cockpit and hoping the plane stays in the air. In clown fish, if Munday is right, the acidic seawater appears to short-circuit the fishes’ sense of smell and hearing, and they swim toward peril. It is difficult to ignore the question of what the rest of us might be swimming toward.
From THE WEIGHT OF NATURE: How a Changing Climate Changes Our Brains by Clayton Page Aldern, to be published on April 9, 2024, by Dutton, an imprint of Penguin Publishing Group, a division of Penguin Random House, LLC. Copyright © 2024 by Clayton Page Aldern.
