If you are new to this series, I’ve been trying to break down the competing claims about genetic engineering. I’m not an expert: When I told a friend I was writing about GMOs he asked, “So are you for them, or against?” My answer: “I’m trying to figure that out.”
The next step in trying to figure that out is to really understand how genetic engineering works. Is this process simply a minor extension of plant-breeding techniques? Or is there a way in which genetic engineering represents a fundamental discontinuity with the age-old practice of farmers selecting seeds?
That’s what I asked Pamela Ronald, a scientist at U.C. Davis who uses genetic engineering to study rice. I approached Ronald because she’s not one of those scientists who is so used to looking through the microscope that she loses sight of the big ecological picture. Her husband, Raoul Adamchack, teaches organic agriculture at Davis, and together they wrote the book Tomorrow’s Table, which makes the case for incorporating genetic engineering into organic practices. Nor is Ronald among the progress-addled optimists who rush to embrace every new technology. She gave birth to her third child in her outdoor hot tub, because the science suggests that — for a normal pregnancy, with one kid already out, and a hospital nearby — it’s actually safer.
When I started to ask questions, Ronald asked if we could back up a bit. “I end up asking people, ‘What is it that bothers you about genetic engineering?’” she said. “Is it the idea of moving genes from one species to another? Well, what we do here is rice — we put rice genes into rice plants. Is it that you don’t like corporations? Well, I’m at a university here, and we’re funded by the government. Is it that you don’t like profits? Well, we have no private funding, and the rice we are developing is all for developing countries. We don’t make money off our discoveries.”
What bothers me about genetically engineered crops, I told her, is that the technology seems to disrupt the co-evolutionary relationship between farmer and plant. I like the idea of farmers saving seeds and coaxing plants toward a greater harmony with their environment (the seasons, the pests, the culture), rather than buying their seeds each year from Monsanto. Plus, in that slow process of selection, it might be easier to weed out any unintended effects that cause problems.
“So,” she said, “in the developed world almost everyone buys their seeds, but the people using our rice can’t afford that. They need to self their seeds” (i.e., they self-pollinate their seeds each year to provide for the next).
In the U.S. farmers buy hybrid seeds, which don’t work as well if you try to save the next generation. But the farmers in Bangladesh, who use rice Ronald pioneered, save seeds every year. The seeds are genetically engineered, yes are breed to contain genes discovered with GE* — but then they continue that process of co-evolutionary selection. As for the risk of unintended problems, Ronald said, “Any time you introduce a new seed there’s some risk, but the risks are small and the benefits are huge. I just think we need to work with whatever technology works best to achieve the goals of sustainable agriculture.”
As with birth, it’s a question of appropriate technology. I wanted to see for myself what it meant to create new seed, and how we might parse the risks of the various methods for doing it. The next day I drove up to Davis. Ronald was traveling, so she left me in the care of Randy Ruan, her lab tech.
Ruan told me to meet him outside the greenhouse. He’d leaned a red bicycle (with an Obama button pined to a pannier) against the glass. He seemed a little bemused by my interest.
“Take as many pictures of rice as you like,” he said.
He had a point. Everything pretty much looked like rice. But the story behind each plant was slightly different.

Rice derived via marker-assisted breeding.
When doing marker-assisted breeding, scientists cross their plants through pollination, hoping to get an exciting new combination of traits. As new plants emerge, they can take a tiny piece of tissue and see if it contains the genes they were hoping for. If not, they can discard the plants. It’s conventional breeding, assisted by a keyhole through which to peek at the DNA.
The problem with conventional breeding, marker-assisted or otherwise, is that it’s messy, said Margaret Smith, a plant breeder at Cornell. (I followed up with her to fill in the nitty gritty of how things worked.) You’re mixing two whole strands of DNA and swapping lots of genes at once, Smith explained. Researchers crossbreed generation after generation with a plant that displays an interesting mutation, creating thousands of plants, most of which they will destroy. It’s not exactly the slow dance with the land that I’d imagined.

This rice was exposed to radioactivity to induce mutations. FN stands for “fast neutron.”
Another way to tweak crops is to induce mutations by dousing seeds in mutagenic chemicals or zapping them with radiation. This causes bits of DNA to copy incorrectly, which causes more changes than you generally see with genetic engineering. “You’re just rolling the dice and hoping to get something interesting,” Smith said.
It works. As it turns out, the 20-pound bag of organic brown rice on top of my refrigerator was a strain (Calrose 76) that mutated after exposure to 25 kR of Cobalt-60 gamma radiation.

Genetically engineered rice.
The most common mode of experimentation in Ronald’s lab, of course, is genetic engineering. Ruan gamely pointed out a few examples. Ronald had mentioned that there were two main projects for which her lab is known: the discovery of the gene XA21, which confers resistance to bacterial disease — good for farmers in the developing world who can’t afford antibacterial pesticides; and a gene that allows rice to tolerate submergence better — good for those same farmers, who now have an herbicide-free way of drowning weeds without drowning the rice.
There are two main ways of genetically engineering plants: shooting them with a gene gun, or using the microbe Agrobacterium tumefaciens. A gene gun literally shoots pellets coated with DNA through plant tissue. As a result of this pure mechanical force, a few genes end up in the nucleus and are incorporated into it. Ronald’s lab, however, uses Agrobacterium. With a little arm twisting, I got Ruan to take me to the lab and walk me through the process.

Mosaics outside the Ronald lab in Robbins Hall.
I wanted to understand in detail how this worked because, years ago, I had attended a lecture given by Ignacio Chapela, a critic of genetic engineering, and his critique had turned on these details. Genetic engineers often make it sound as if they are cutting and pasting DNA in precise places, he said, but the genes are sprayed into the genome at random. The thing that really bothered Chapela is that scientists bundle the gene they want with several others: They will build a sequence starting with a promoter (or “on switch”), then the gene they want to transfer, then a marker (which displays some visible trait to show them everything is working), and a terminator (the “off switch”).
Throw all this at a genome and it could cause trouble: The terminator sequence could break off, Chapela pointed out, and all of a sudden the plant is expressing not just the trait you want, but also whatever comes right after that in the genome. Plants often have inactive genes for the manufacture of toxins, for instance, and the randomness of genetic engineering could turn them on.
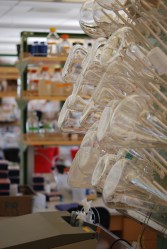
Flasks in Ronald’s lab.
All this, it turns out, is absolutely true. But it’s also occurring all the time in the wild and in plant breeding, without the assistance of genetic engineering. The process for building the bundle of genes is, in actuality, incredibly precise. Because researchers are working with a relatively small amount of DNA, they really can cut and paste with precision. To this sequence, they add a bit of DNA called a plasmid — which catches both ends of the sequence, turning it into a circle. Plasmids are strange and fascinating things: They are essentially tools that bacteria use to swap genetic information between species — an instrument for creating transgenics built by evolution.

A tray of plasmids carrying DNA bundles in a freezer.
Next comes Agrobacterium. This particular microbe specializes in injecting plasmids into plant DNA. In the wild it does this with genes that make the plant form a home in which the Agrobacterium thrive. Scientists simply replace those plasmids with the ones they’ve constructed.
Chapela was correct to say that this part of the process is random; there’s no control over where the Agrobacterium insert their payload, and there is a chance that this bundle of DNA can fracture. But, Smith told me, the same thing happens during normal breeding. The promoter might, certainly, turn on unwanted genes. But the promoter, which almost always comes from the cauliflower mosaic virus, is doing the same thing all the time in the wild.
The difference, Chapela had hypothesized, was that genetic engineering methods would lead genes to fall into more vulnerable and unstable sections of the genome. But that hasn’t happened. Analyses of thousands of genomes show that introduced genes fall randomly amid the DNA strands. The genes introduced by humans have proven to be no more likely to break up or move around the genome. (I’m not getting to Chapela’s main point, that engineered genes were spreading with pollen. More on that later.)
Of course, Chapela’s objection was just one possible scenario — others have and will continue to be raised. The point is, it’s easy to overestimate the risk of the new while underestimating the risks of the status quo. Species appear to be fairly stable, but beneath the surface, we live in a churning ocean of genetic flux.
In 2003, when the United Kingdom’s GM Science Review Panel (chaired by climate hawk Sir David King) looked closely at this issue, it concluded that genetic engineering was no more likely [PDF] to produce unintended consequences than conventional breeding:
Conventional plant breeding can produce gross undirected and unpredictable genetic changes and in that sense has considerable uncertainty. This is well documented and we know much about the types of change at a cellular level.
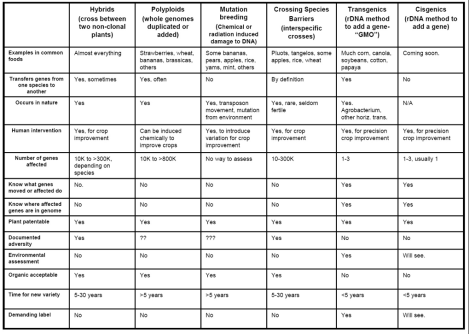
Kevin FoltaClick to embiggen. A handy table comparing forms of genetic modification.
There is, of course, one potentially important difference:
A special feature of GM breeding is that it allows the transfer into crop plants of one or a few genes from what might be radically different organisms. Conventional breeding cannot, for example, form plants that can assemble complex human immunoglobulins as has been achieved in GM plants. This inevitably raises uncertainty about whether there are any novel genetic interactions and whether these are potentially harmful …
A further special feature of GM breeding is that the products of particular gene constructs may become present in radically different foodstuffs, effectively independently of any biological relationships … this can hold important implications for risk management policy in areas such as the avoidance of exposures to any allergens that might pass through regulatory screening.
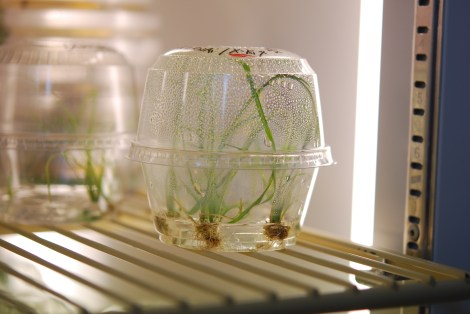
Recently engineered rice sprouting.
As a result, genetically engineered foods are screened for potential allergens. It’s frequently pointed out that Pioneer Hi-Bred mistakenly introduced an allergen into soybeans when it added a gene from Brazil nuts. The rest of the story is that we know about this because there was the right testing regime, and the product never went on the market — the company (and the regulators) knew what to look for and successfully weeded the plant out.
So what’s the takeaway of all this? Before I finished up my conversation with Margaret Smith, I asked her if there might be some evolutionary wisdom in the way genetic material gets swapped during normal reproduction that was fundamentally different than techniques of genetic engineering. We don’t know of any, she said. But she added:
I think we need to be thoughtful, and as we learn more we need to continue to think about this carefully. We’re learning more every day — just look at the revolution in epigenetics — and that could change the way we approach this. But my message on this is that we shouldn’t just stop because there are unknowns. Every technology has unknowns. We just have to be as thoughtful as we can.
Those of us who are suspicious of genetically engineered foods need to be thoughtful, too. It makes no sense, for instance, to protest GMOs while accepting that irradiated organic mutants should be exempt from any special regulation. It makes no sense to try to ban all genetically engineered foods if we aren’t concerned about the rice-to-rice transfers that people like Ronald are doing.
I still think that we have an important role to play in making sure the technology isn’t used inappropriately. But it’s not useful to flail blindly against something we don’t understand.
Update: Pamela Ronald made it clear during our initial conversation that, while she used genetic engineering for gene discovery, it was her collaborators, using marker-assisted selection who actually developed rice for farmers. I omitted this because I thought that a discussion of the distinction between basic and applied science would be tangential to the main point: How is GE different from conventional breeding?
This distinction, however, raises another important question, namely: Is genetic engineering actually a useful tool for sustainable agriculture? I’ll be getting to that.
I did make one real mistake. Ronald’s lab found the submergence tolerance gene Sub1, which is indeed the gene that was released in the Bangladeshi varieties, but it was introduced through marker-assisted selection. I regret the error.
More in this series:
- The genetically modified food debate: Where do we begin?
- The GM safety dance: What’s rule and what’s real
- Is nature a cradle or a battlefield?
- Genetically engineered food: Allergic to regulations?
- Genetically modified seed research: What’s locked and what isn’t
